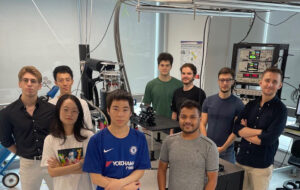
NUS Materials Science and Engineering Asst. Prof. Denis Bandurin’s research team at CMX-lab has made significant advancements in terahertz-enabled sample characterisation. They have developed a novel approach that intertwines conventional low-temperature magnetotransport measurements with intricate electronic effects, actuated by the incident terahertz radiation. The immense potential of this method was unveiled during the testing on the nanostructure with an artificially engineered moiré superlattice formed on the interface between a pair of two-dimensional materials: bilayer graphene and hexagonal boron nitride. The experimental findings revealed complex electronic band structure with peculiar topological properties that so far remained elusive for conventional measurement techniques.
One of the surprising discoveries — a giant magnetic moment carried by electrons, which is essentially a tiny nanoscale magnet confined within a particle. The remarkable aspect lies in its purely quantum nature, which arises from the interference between the lattice-defined configuration of the electronic wave packets and the non-trivial topology of the band structure. This renders magnetic moment as a useful tool for exploring Berry-curvature hot spots, where the quantum fabric of the band structure’s geometry stretches and bends. The challenging part remains the detection of the magnetic moment, as it typically does not reveal itself in transport measurements. In the setup, however, electronic magnetic moment leads to a pronounced valley symmetry breaking, which manifests in a strong valley-splitting of photovoltage signal built up under finite magnetic field and incident terahertz light.
In the ACS Nano papers, the researchers also report on how the scope of terahertz applications may include the research of the microscopic transport in graphene-based structures. Their approach offers a powerful alternative to the commonly used method that relies on the analysis of the temperature-dependent resistivity behaviour and requires setting the sample’s temperature to the temperature of the cryostat. This requirement makes it challenging to disentangle the origin of processes that cause the enhancement of resistivity whenever its temperature trend deviates from the expected textbook pattern. This issue is particularly relevant to the devices featuring moiré superlattices, as they exhibit odd temperature dependencies, lacking straightforward interpretation. The aforementioned problem can be circumvented by using terahertz radiation as a power source to energies carriers in graphene. Owing to the extremely slow electron thermalization, graphene’s lattice remains cold under the terahertz beam, while vanishingly low specific electronic heat of graphene enables substantial overheating of electrons even by minute energy inputs. Overall, the lattice and electron temperatures become effectively detached, allowing unambiguously analyse on their role in the graphene transport.
Looking ahead, the research team plans to extend the application of the developed approach to more complex physical systems and expand the frequency range of the radiation sources used to probe their properties. Their findings advance the understanding of phenomena introduced by moiré superlattices and unlock a new chapter in physics of low-dimensional nanostructures.
To read more, visit: https://pubs.acs.org/doi/10.1021/acsnano.3c13212