RESEARCH
Island biodiversity and Jane Jacobs' urban vision
Dr Andrew Schauf (Research Fellow, NUS Cities) exemplifies the interdisciplinary approach of NUS Cities, applying insights from evolutionary biology to discuss whether high-density cities are a boon or bane for humanity.
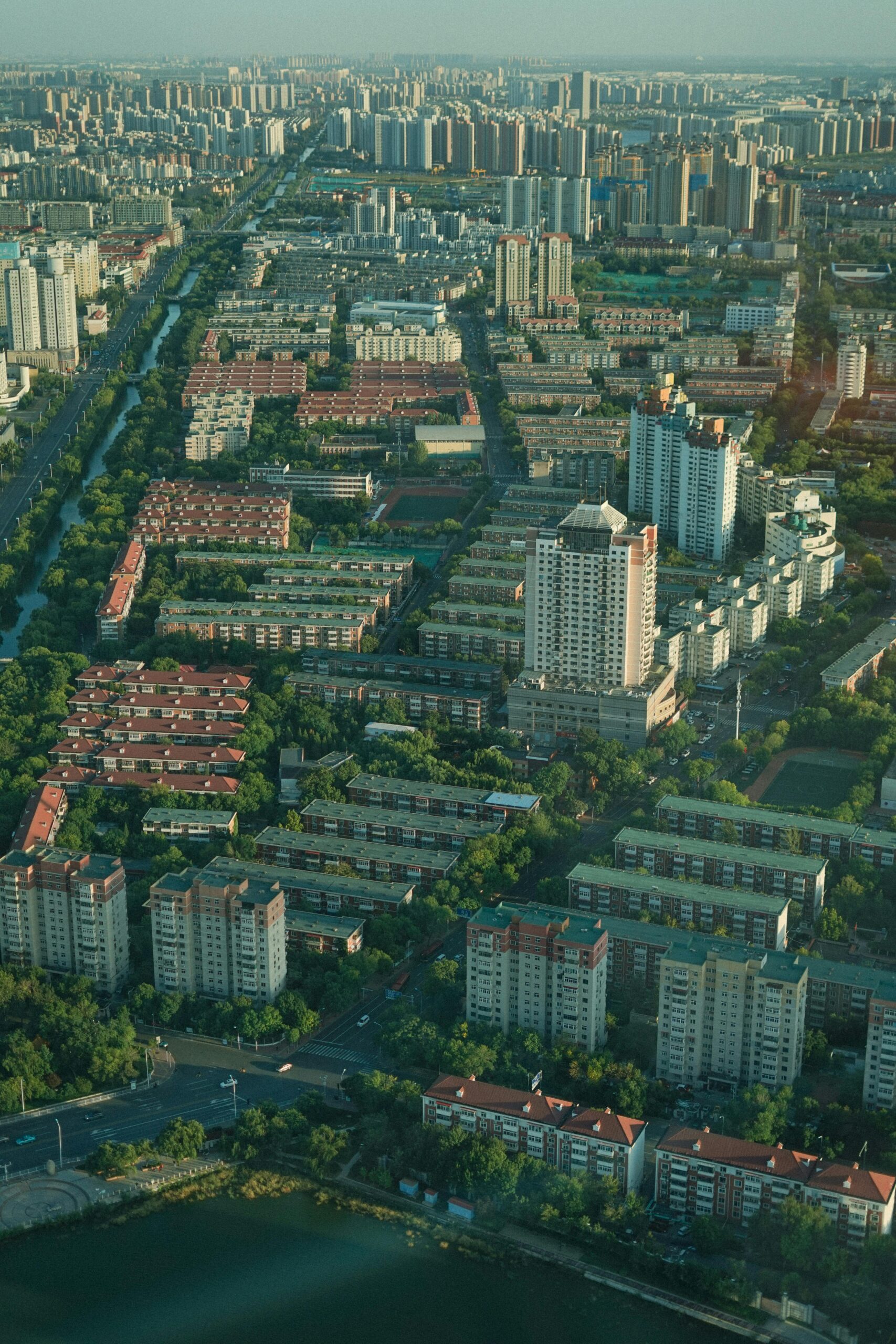
Does higher density support well-being in cities? No. But also, yes. It’s complicated.
Utopian urbanists reacting to the squalor of overcrowded nineteenth-century cities answered ‘no’. Frank Lloyd Wright envisioned “a minimum of an acre per person” [1].
Certain high-modernist designers were a bit more ambivalent. Aiming to “reconcile the seeming opposites of urban design: density and open space” [1], Le Corbusier called for blocks of “fantastically high density”, but arranged them on grids separated by vast distances so that “95% of the ground could remain open” [2].
Jane Jacobs, though, gave an emphatic ‘yes’. An entire chapter of The Death and Life of Great American Cities was devoted to “The Need for Concentration” [2] . Rejecting the “rigid segregation” [3] of high-modernist city plans, she also contextualized this need within a more multifaceted notion of diversity; a “dense concentration of people” was just one of Jacobs’ “four conditions of generating diversity” [2]. The “supposed correlation between high densities and trouble”, Jacobs insisted, was “simply incorrect”. Nor was any single factor sufficient in isolation; “[a]ll four [conditions] in combination are necessary”, and “the absence of any one” factor can suffocate vitality [2] .
For me, Jacobs’ descriptions conjure up mental images of a landscape overlaid with multiple density maps, each representing a distinct aspect of the urban fabric (say, residential population, businesses, green spaces, or other amenities). Over time, each of these density maps evolves—perhaps growing here, while thinning out over there—according to its own logic. Crucially, though, these densities are highly coupled to one another; changes to one will trigger changes in the others as they co-evolve in space. So, the same basic elements, if positioned and combined in different ways, could evolve towards completely different outcomes in the long run.
Small-scale choices about how to allocate and combine these components at the street level could then have drastic, widespread consequences. Indeed, “Jacobs begins with informal, social order from below” rather than “architectural order from above”, urging us to recognize “the city as a social organism” whose “interconnections are so complex ... that planning always risks unknowingly cutting into its living tissue, thereby damaging or killing” it [3] . Far from disempowering planners, though, this suggests that a thoughtful placement of those few “chess pieces” [2] that planners can control could have huge impacts.
Given these images of cities as living systems, it seems germane to wonder how a biologist might answer our question: Does higher density support well-being?
But again, it’s complicated. When an environment is pushed to the limits of its carrying capacity, then competition for scarce resources decides who survives and who dies. Density can kill.
Elsewhere, though, countless factors make higher density beneficial. Biologists call these Allee effects. Animals find strength in numbers while foraging, hunting, or defending themselves [4]. Plants can too; whereas a lone seedling might die from exposure, higher vegetation densities can provide protection, water retention, and other forms of ‘positive feedback’ that support further growth [5]. Spatial aggregation—not isolation—becomes preferable. The same can hold for reproduction. Animals in sparsely populated areas might fail to find mates; pollen scattered by an isolated plant may fall short of its nearest neighbor. Mate-finding Allee effects [6] are then at play. These make population dynamics highly sensitive to density, as in cities [7]. Two otherwise-identical populations, if scattered differently through space, can evolve to have drastically different characteristics.
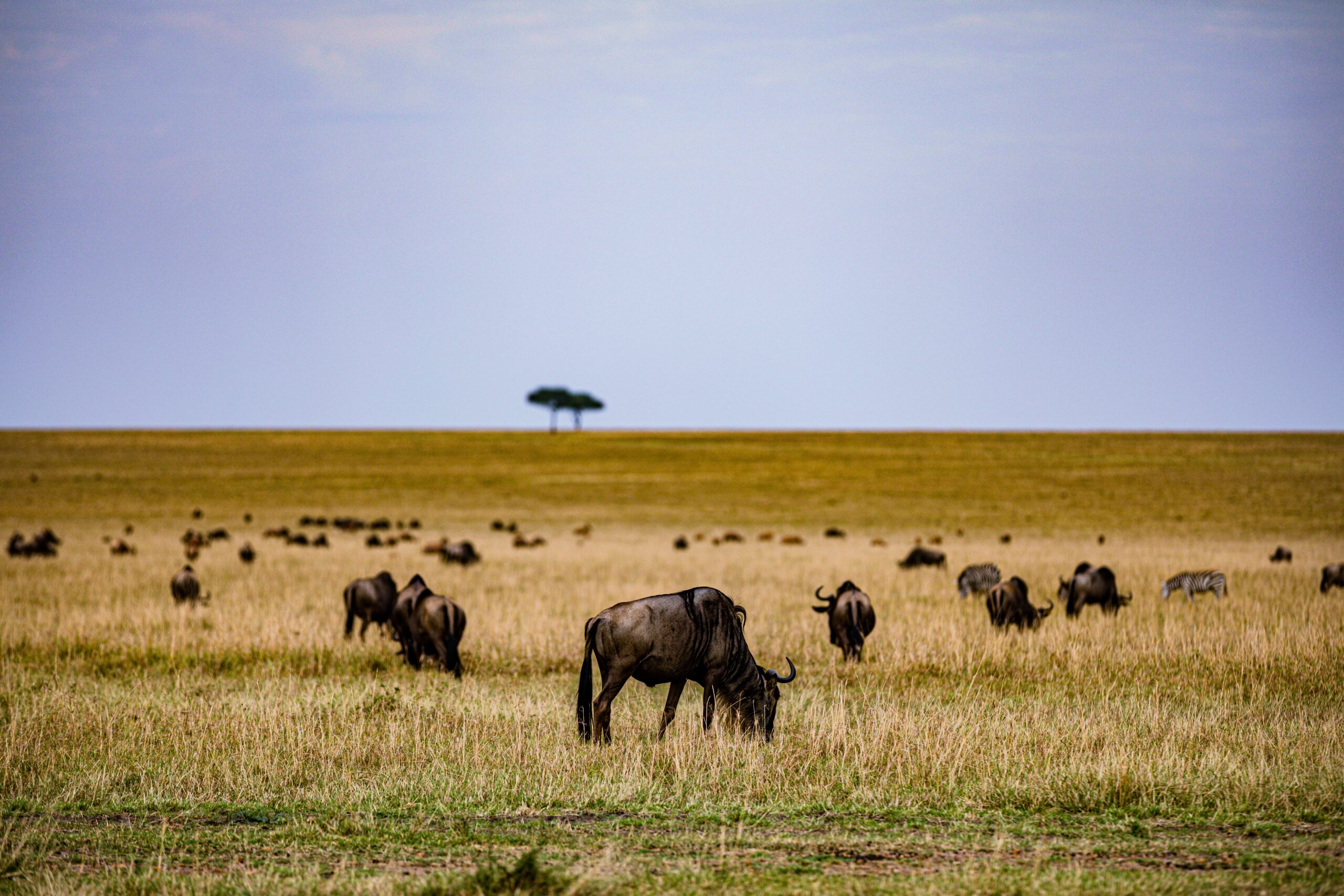
I recently explored this idea in a collaboration with Matt Jones and Poong Oh [8]. I first bonded with Matt over dinosaurs at age four; unlike me, he stayed on track and became a paleontologist. While I tagged along on his expedition to Malaysia, our discussions about nusantara biodiversity gave rise to a question: How do different aspects of a population’s spatial fragmentation (i.e., the sizes and densities of separate population patches, and the distances between them) shape the characteristics of a population as it evolves under mate-finding Allee effects?
The more we pondered, the more we found ourselves visualizing something like the overlapping density maps I imagined while reading Jane Jacobs. A diverse population can be seen as a set of overlapping sub-populations, each with its own dispersal ability (a number describing the speed of their ‘random walk’ wanderings). Each sub-population spreads at its own rate, but meanwhile—as with urban densities—these multiple layers are constantly interacting (here, through mating). In Jacobs’ accounts, precariously low densities of one component of urban diversity can sometimes be resurrected with help from other, more stable components; here too, low densities of one vulnerable sub-population can be ‘genetically rescued’ [9] through mating with other groups.
This matters most for the fast-moving pioneers who lead the charge into unpopulated regions. By doing so, they bear the brunt of the negative effects of low density. In the process, though, they increase population densities there, protecting future generations from those effects. Their fate depends on the sizes of the unpopulated areas they must traverse, and also their proximity to slower-moving peers who can ‘genetically rescue’ them. So, the initial arrangement of populated and empty regions in space can determine the balance of ‘fast’ versus ‘slow’ genes that live on. The trade-offs involved here recall the urban planner’s task of balancing “density and open space”.
If you were an ecological ‘planner’ tasked with allocating a population throughout a habitat, how would you balance these? Would groups of densely-packed, isolated clusters best ensure survival and diversity? Or should you spread them thinly to cover more ground? Imagine you had to choose the spatial range of an intervention that would wipe out a population across its target area (Conservation ecologists might hope to protect a threatened species; for invasive species, they might aim for the opposite outcome). What should you do?
To address these kinds of questions, we pictured fragmented populations in an idealized way: as grids of identical patches (looking not unlike a high-modernist town plan; see Figures 1 & 2). We then simulated how these populations would spread, inter-breed, and evolve different dispersal characteristics depending on their initial fragmentation patterns.
Many previous models predicted that environmental diversity inevitably drives dispersal abilities downward. Our model captured a situation where dispersal ability can sometimes evolve upward along advancing population fronts, as has been documented for invasive species like cane toads [10]. Most related models also suggest that a population’s initial arrangement in space has no effect on its long-term outcomes. By contrast, we presented two arrangements of the same total population, under the same environmental conditions, whose dispersal abilities evolved in completely different directions: one upward, one downward (see Figure 3). In this way, we illustrated a ‘path-dependent’ situation where—as in cities—long-term diversity and vitality can be highly contingent on how a population’s various “chess pieces” are initially arranged in space.
It seems natural, then, that this class of models used in ecology [11] has also found applications to urban morphology [12] and traffic [13]. Please don’t tell the editors at Ecology and Evolution, but in retrospect it seems to me that Jane Jacobs did it first.
The black-and-white diagram (Figure 1) may recall certain idealized town plans like Ebenezer Howard's "Garden City" (Figure 2). Here, though, they represent a simplified version of the "fragmented" populations that form when multiple clusters of a species colonize a new habitat, or when an established population is partially wiped out by a disaster.
By varying the details of this basic layout (s: the spacings between patches, r: the sizes of the patches, and ρ: their population densities), we explored how the initial spatial arrangement of a population shapes the characteristics it then evolves as its members wander around, mate, and die over many generations.
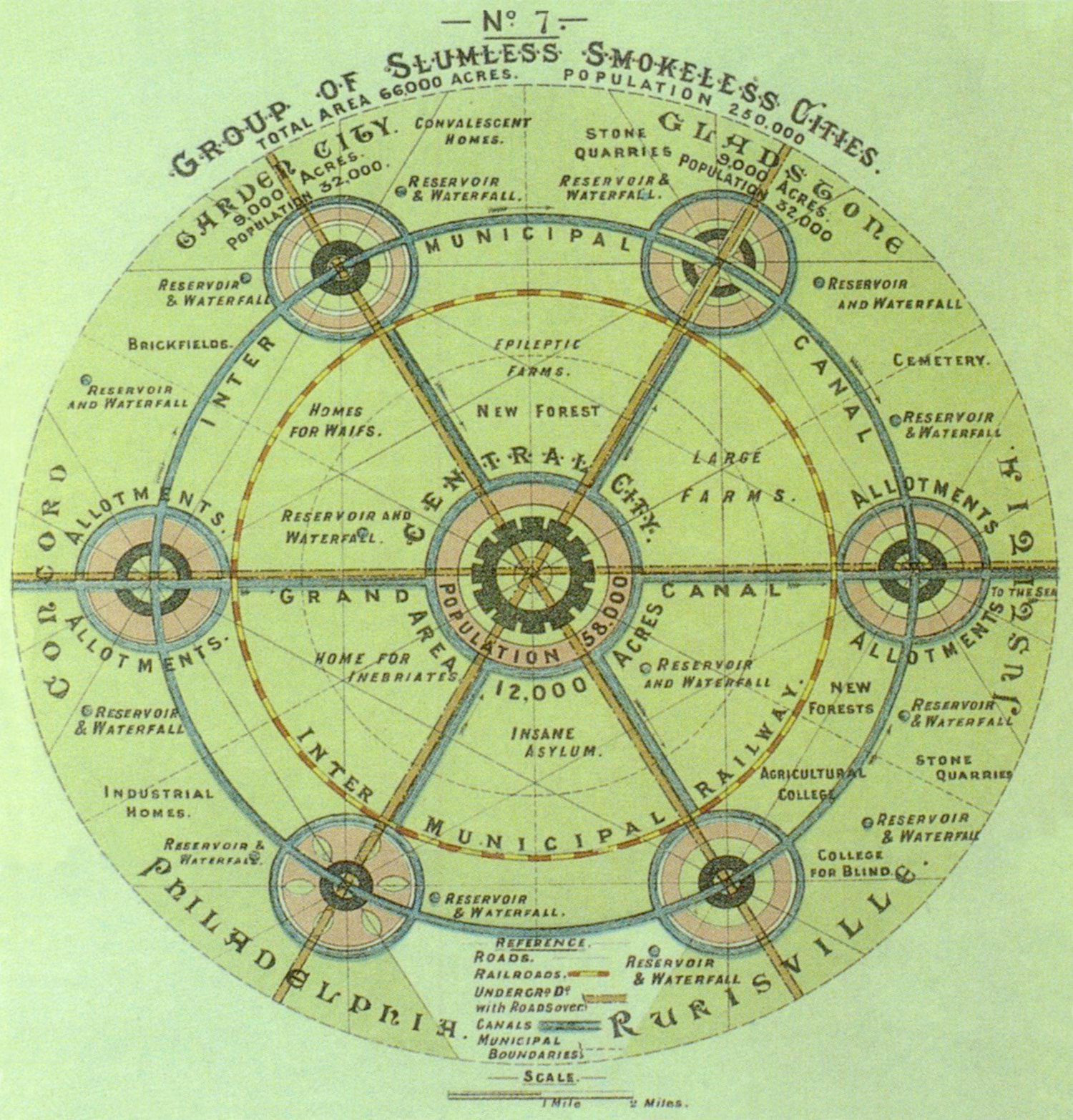
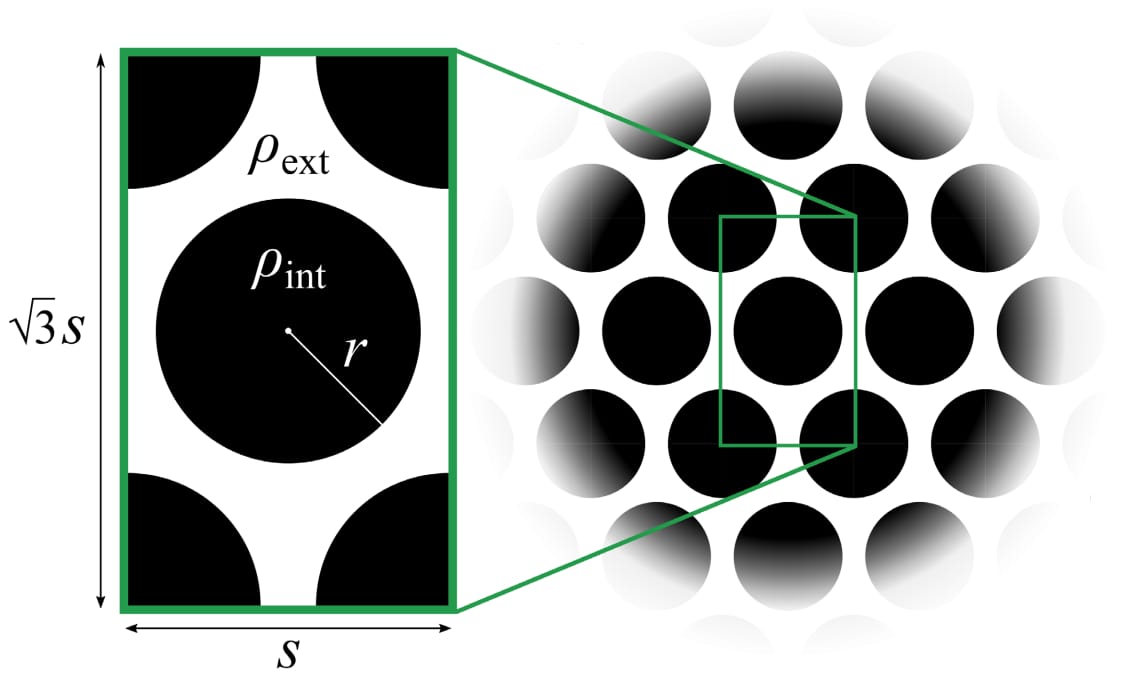
Like many complex urban systems, our ecological model exhibits strong "path dependency"; its outcomes depend on the details of how things start out. Where Allee effects act, the same total population can evolve towards very different outcomes, depending on how it is arranged in space: for one arrangement, slower-moving members of the population come to dominate (left column of Figure 3; 'Coarse fragmentation'); for another, faster movers win out (right column of Figure 3; 'Fine fragmentation'). Both arrangements are "self-similar"—made up of big fragments which are themselves made up of similar smaller fragments—but differ in terms of small fragment sizes.
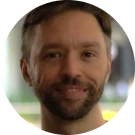
Dr Andrew Schauf, Research Fellow with NUS Cities, has a background in Physics and Applied Math. To find out more about his work on Allee effects, please read his journal article: Simulating the dynamics of dispersal and dispersal ability in fragmented populations with mate‐finding Allee effects
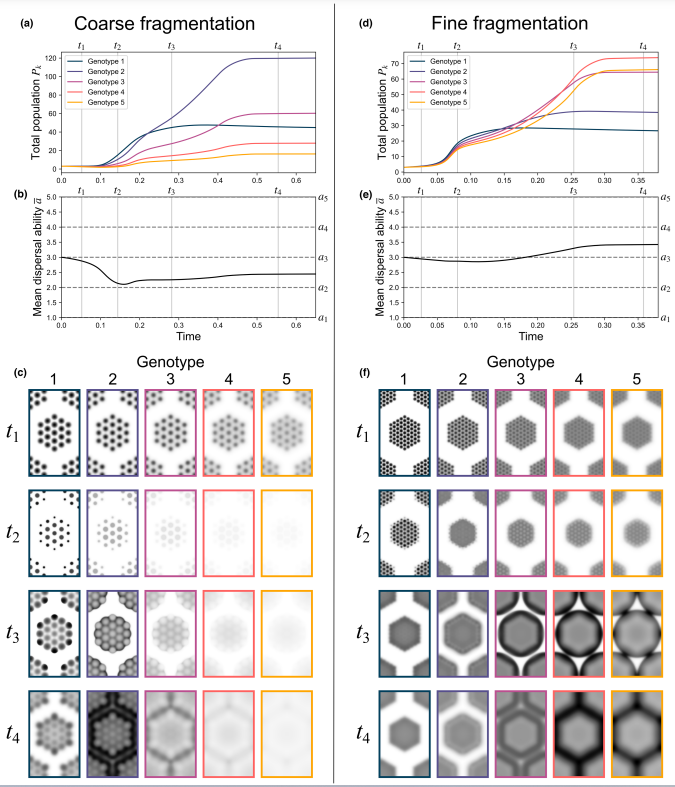
References
[1] Fishman, R. (1982). Urban Utopias in the Twentieth Century: Ebenezer Howard, Frank Lloyd Wright, Le Corbusier. MIT Press.
[2] Jacobs, J. (2011). The Death and Life of Great American Cities: 50th Anniversary Edition. Random House.
[3] Scott, J. C. (2020). Seeing Like a State: How Certain Schemes to Improve the Human Condition Have Failed. Yale University Press.
[4] Kropotkin, P. (2013). Mutual Aid: A Factor of Evolution: University Edition. Sotina Publishing.
[5] Scheffer, M. (2020). Critical Transitions in Nature and Society. Princeton University Press.
[6] Gascoigne, J., Berec, L., Gregory, S., & Courchamp, F. (2009). Dangerously few liaisons: A review of mate-finding Allee effects. Population Ecology, 51, 355-372.
[7] Before I push the urban ‘Allee effects’ analogy too far, note that some influential hot takes on cities seem to blur the distinctions between the ‘positive’ vs. ‘negative’ effects of higher density, or even ‘cooperation’ vs, ‘competition’. Some argue that urban density is useful precisely because it forces different groups into conflict over contested space and resources (with the important caveat that none of these groups feel thereby threatened with ‘extinction’. See: Sennett, R. (2021). The Uses of Disorder: Personal Identity and City Life. Verso Books.).
[8] Schauf, A. J., Jones, M. F., & Oh, P. (2023). Simulating the dynamics of dispersal and dispersal ability in fragmented populations with mate‐finding Allee effects. Ecology and Evolution, 13(4), e10021.
[9] Whiteley, A. R., Fitzpatrick, S. W., Funk, W. C., & Tallmon, D. A. (2015). Genetic rescue to the rescue. Trends in Ecology & Evolution, 30(1), 42-49.
[10] Phillips, B. L., Brown, G. P., & Shine, R. (2010). Evolutionarily accelerated invasions: The rate of dispersal evolves upwards during the range advance of cane toads. Journal of Evolutionary Biology, 23(12), 2595-2601.
[11] Cantrell, R. S., & Cosner, C. (2004). Spatial Ecology via Reaction-Diffusion Equations. John Wiley & Sons.
[12] Friesen, J., Tessmann, R., & Pelz, P. F. (2019, May). Reaction-diffusion model describing the morphogenesis of urban systems in the US. In GISTAM (pp. 88-96).
[13] Bellocchi, L., & Geroliminis, N. (2020). Unraveling reaction-diffusion-like dynamics in urban congestion propagation: Insights from a large-scale road network. Scientific Reports, 10(1), 4876.